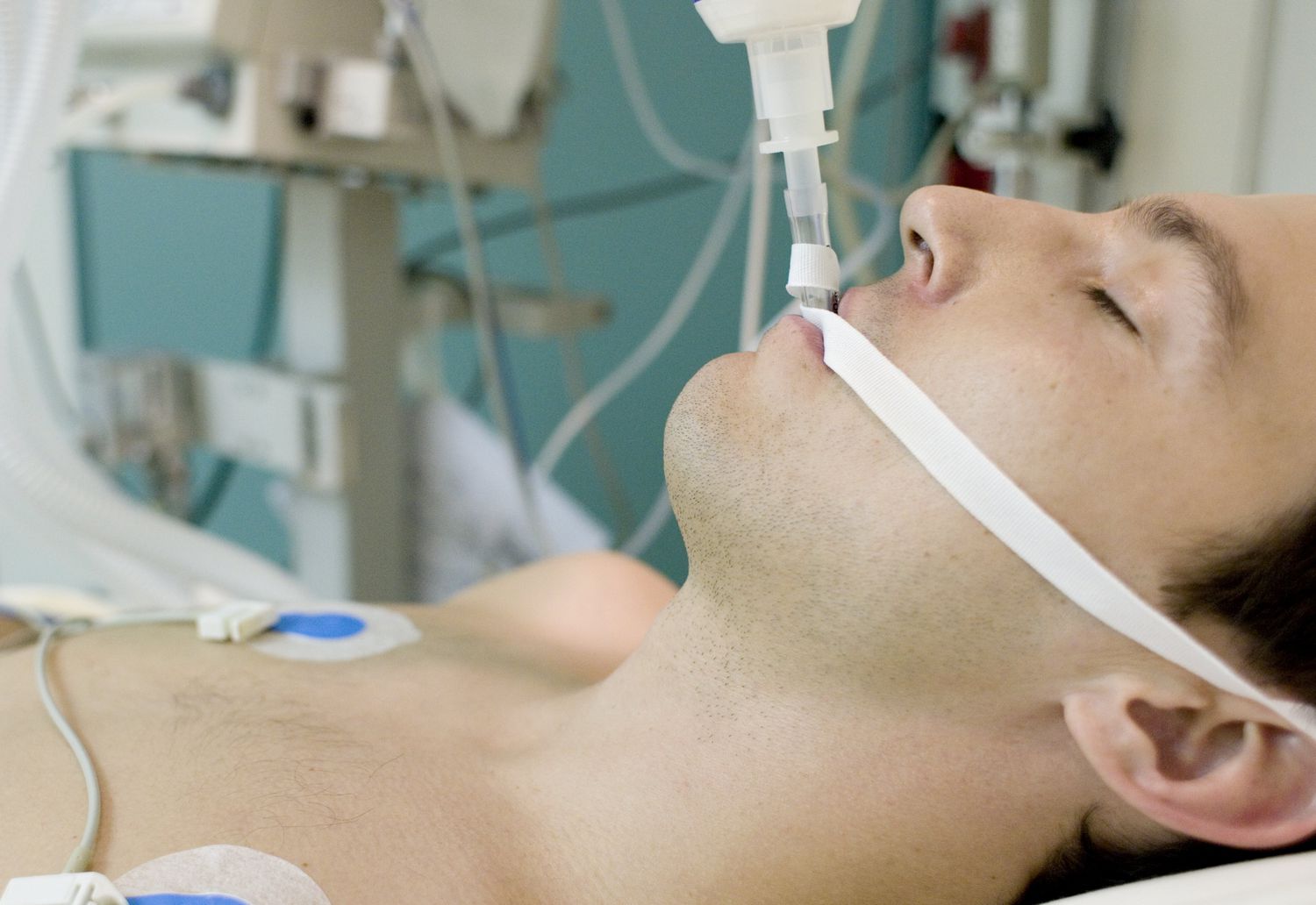
Practice Essentials
Metabolic acidosis is a clinical disturbance characterized by an increase in plasma acidity. Metabolic acidosis should be considered a sign of an underlying disease process. Identification of this underlying condition is essential to initiate appropriate therapy. The initial therapeutic goal for patients with severe acidemia is to raise the systemic pH above 7.1-7.2, a level at which dysrhythmias become less likely and cardiac contractility and responsiveness to catecholamines will be restored.
This article discusses the differential diagnosis of metabolic acidosis and presents a scheme for identifying the underlying cause of acidosis by using laboratory tests that are available in the emergency department. Clinical strategies for treating metabolic acidosis are also reviewed.
Signs and symptoms of metabolic acidosis
Metabolic acidosis can result in a variety of nonspecific changes in several organ systems, including, but not limited to, neurologic, cardiovascular, pulmonary, gastrointestinal, and musculoskeletal dysfunction. Symptoms are often a result of and specific to the underlying etiology of the metabolic acidosis.
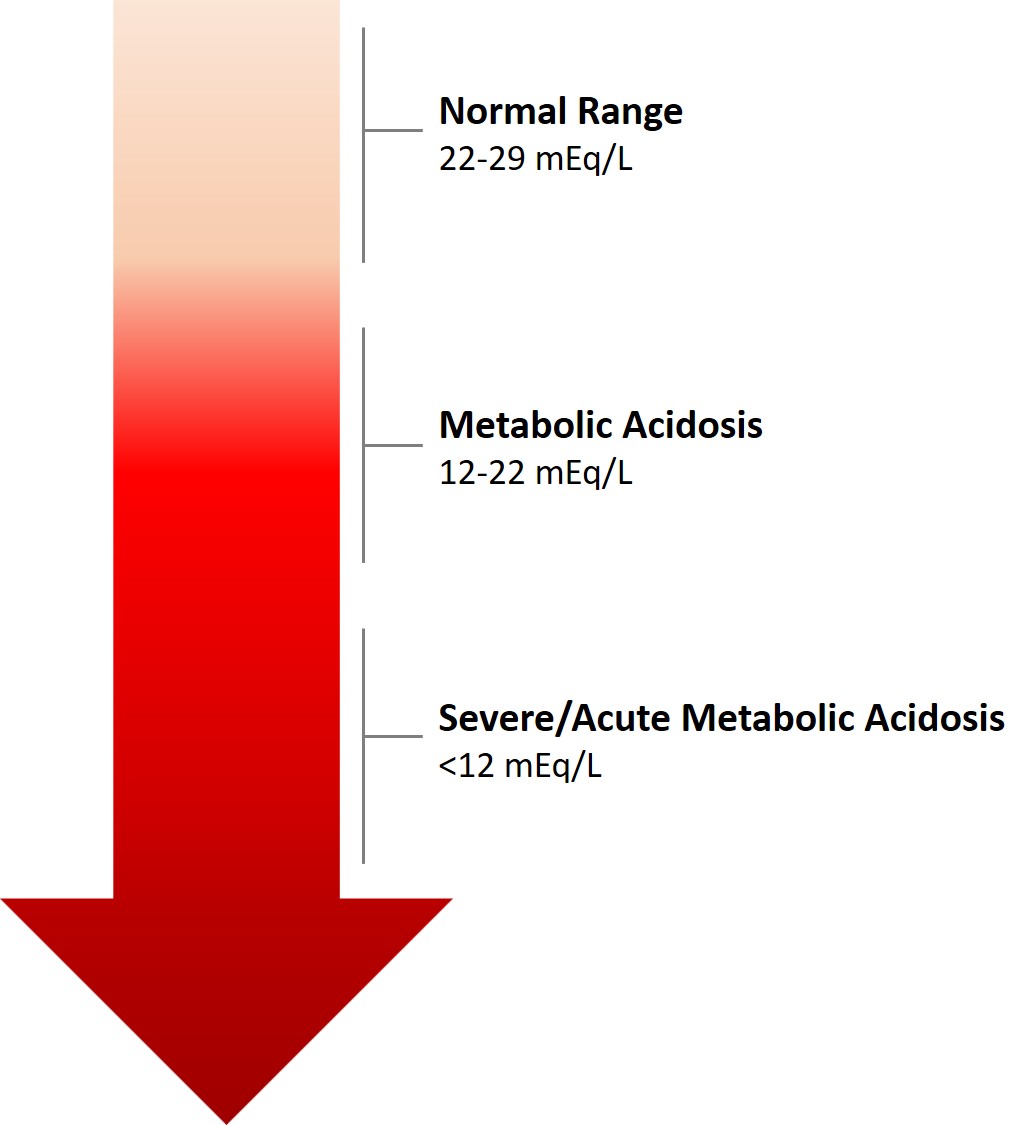
Neurologic signs and symptoms include the following:
- Cranial nerve palsies may occur with ethylene glycol intoxication
- Retinal edema may be seen in methanol ingestions
- Lethargy, stupor, and coma may occur in severe metabolic acidosis, particularly when it is associated with a toxic ingestion
With regard to the cardiovascular system, severe acidemia (ie, pH < 7.10) can predispose a patient to potentially fatal ventricular arrhythmias, and it can reduce cardiac contractility and the inotropic response to catecholamines, resulting in hypotension and congestive heart failure.
Pulmonary signs and symptoms include the following:
- Patients with acute metabolic acidosis demonstrate tachypnea and hyperpnea as prominent physical signs
- Kussmaul respiration, an extremely intense respiratory effort, may be present
- Hyperventilation, in the absence of obvious lung disease, should alert the clinician to the possibility of an underlying metabolic acidosis
With regard to the musculoskeletal system, chronic metabolic acidosis (eg, uremia, renal tubular acidosis [RTA]) is associated with substantial bone disease from bone buffering of calcium carbonate.
Workup in metabolic acidosis
Lab studies in the workup of metabolic acidosis include the following:
- Arterial blood gas analysis – A low HCO 3 level found on an automated sequential multiple analyzer (SMA) (eg, serum chemistries) is often the first clue to the presence of metabolic acidosis
- Serum chemistry
- Complete blood count (CBC)
- Urinalysis – If the urine pH is above 5.5 in the face of acidemia, this finding is consistent with a type I RTA
Other tests include the following:
- Anion gap (AG)
- Ketone levels
- Serum lactate levels
- Salicylate levels
- Iron levels
Management of metabolic acidosis
The initial therapeutic goal for patients with severe acidemia is to raise the systemic pH above 7.1-7.2, a level at which dysrhythmias become less likely and cardiac contractility and responsiveness to catecholamines will be restored.
Treating the underlying conditions in high AG states usually is sufficient to reverse the acidosis. Treatment with bicarbonate is unnecessary, except in extreme cases of acidosis when the pH is less than 7.1-7.2. For all cases of diabetic ketoacidosis, the role of bicarbonate is controversial, regardless of the pH or bicarbonate level.
In hyperchloremic acidosis, the central problem is with the reabsorption or regeneration of bicarbonate. In these conditions, therapy with bicarbonate makes physiologic sense and is prudent in patients with severe acidosis.
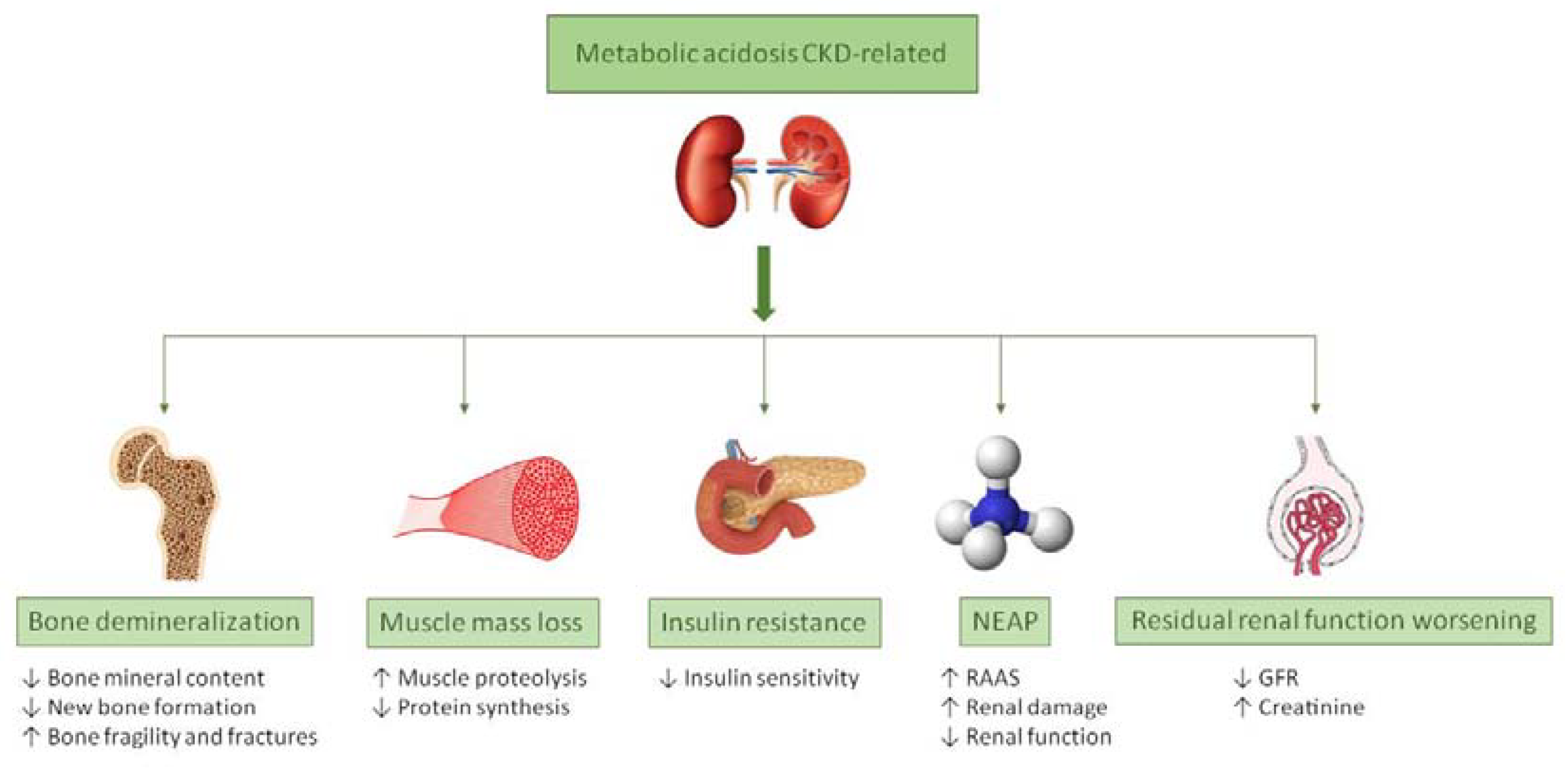
Pathophysiology
There are 3 approaches to understanding acid/base balance: A qualitative approach using the Henderson/Hasselbalch equation, a semiqualitative approach with base excess, and the Strong Ion Theory. The 3 theories are reviewed below.
Henderson-Hasselbalch approach to acid/base physiology
The Henderson-Hasselbalch equation describes the relationship between blood pH and the components of the H2CO3 buffering system. This qualitative description of acid/base physiology allows the metabolic component to be separated from the respiratory components of acid/base balance.
pH = 6.1 + log (HCO3/H2CO3)
Bicarbonate (HCO3) is in equilibrium with the metabolic components.
Bicarbonate production in the kidney
Acid production from endogenous or exogenous sources
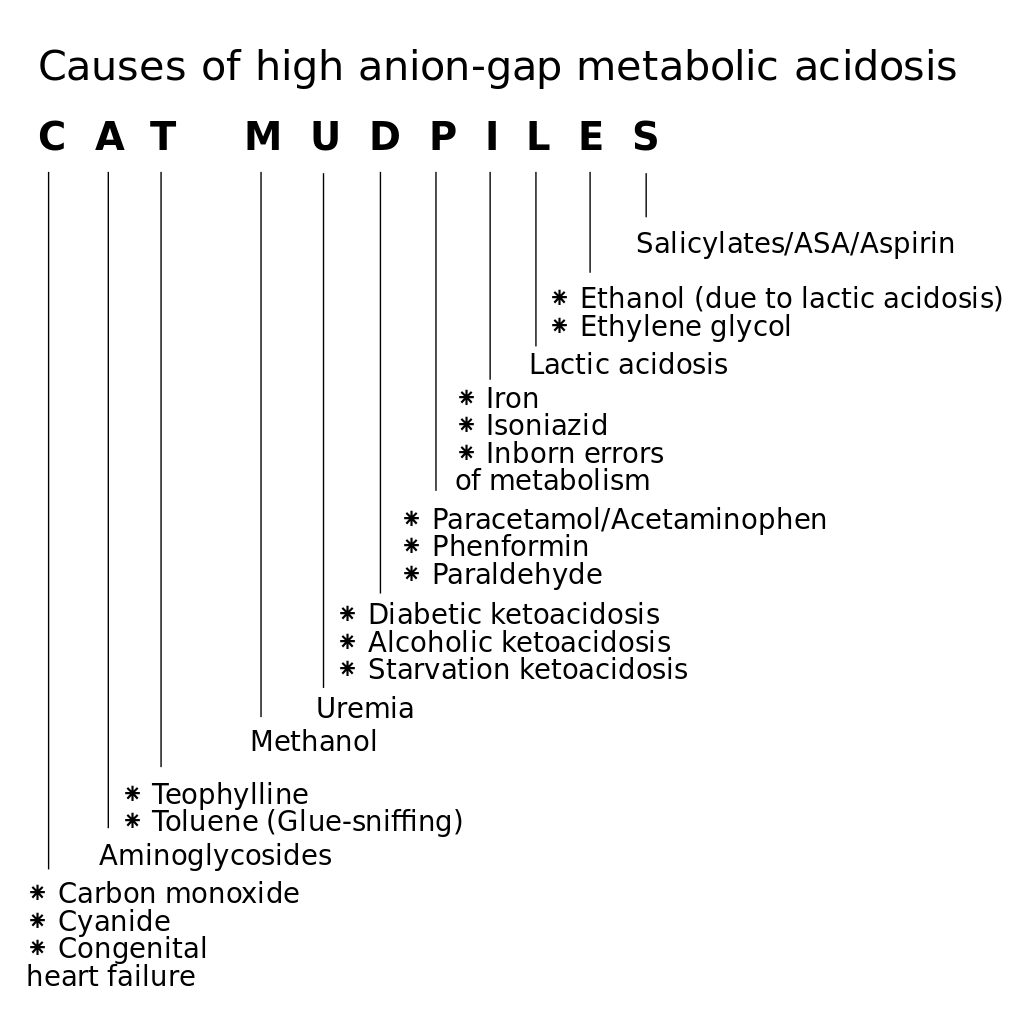
Carbonic acid (H2CO3) is in equilibrium with the respiratory component, as shown by the below equation:
H2CO3 = PCO2 (mm Hg) X 0.03
Metabolic acidosis can be caused by the following:
Increase in the generation of H+ from endogenous (eg, lactate, ketones) or exogenous acids (eg, salicylate, ethylene glycol, methanol)
Inability of the kidneys to excrete the hydrogen from dietary protein intake (type I, IV renal tubular acidosis)
The loss of bicarbonate (HCO3) due to wasting through the kidney (type II renal tubular acidosis) or the gastrointestinal tract (diarrhea)
The kidneys’ response to a respiratory alkalosis
Base excess approach to acid/base physiology
Unfortunately, the Henderson/Hasselbalch equation is not linear; pCO2 adjusts pH as part of the normal respiratory compensation for acid/base derangements. This nonlinearity of Henderson-Hasselbalch prevents this equation from quantifying the exact amount of bicarbonate deficit in a metabolic acidosis. This observation led to the development of a semiquantitative approach, base excess (BE).
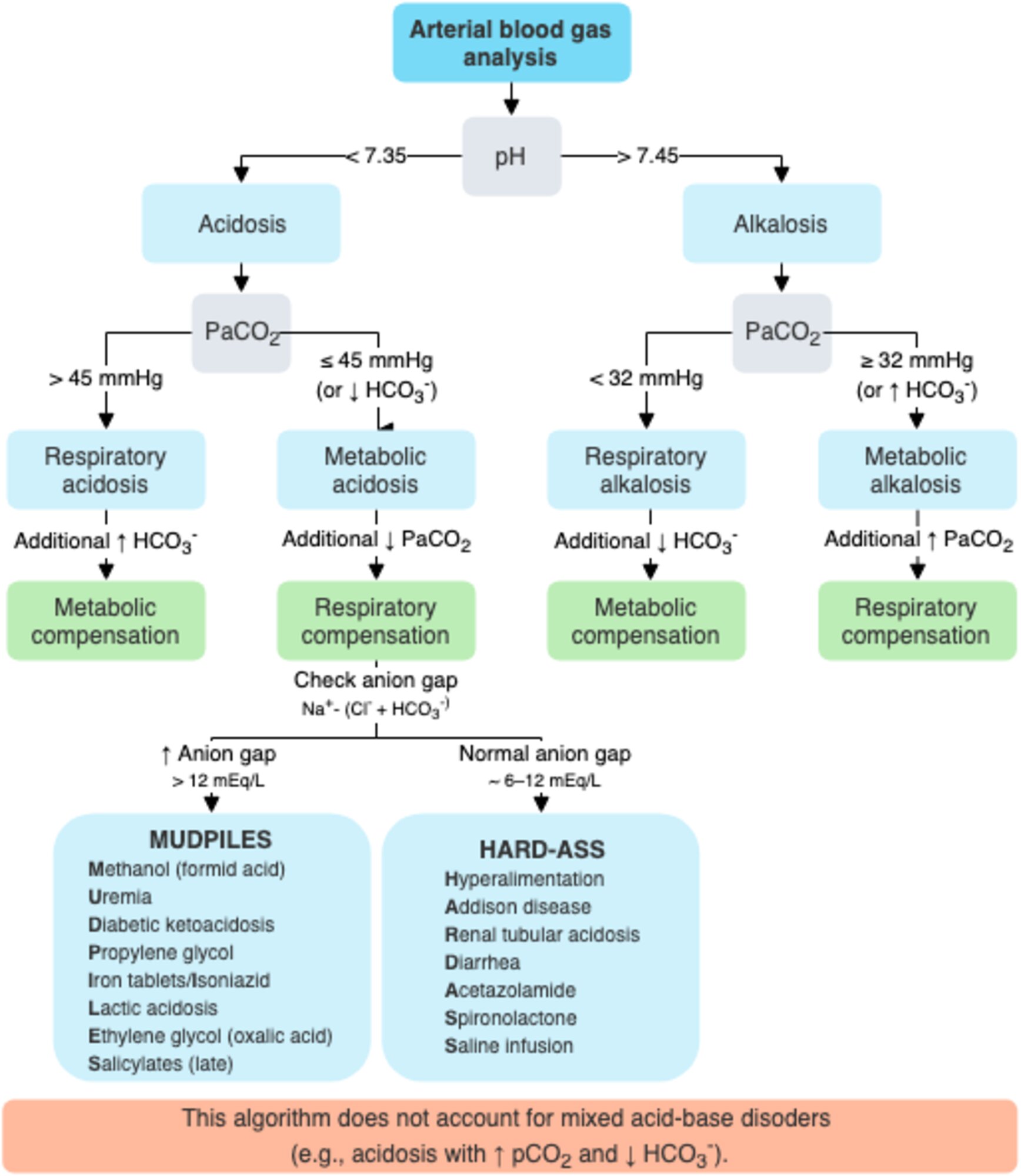
BE = (HCO3 – 24.4 + [2.3 X Hgb + 7.7] X [pH – 7.4]) X (1 – 0.023 X Hgb)
Base excess attempts to give a quantitative amount of bicarbonate (mmol) that is required to be added or subtracted to restore 1 L of whole blood to a pH of 7.4 at a pCO2 of 40 mm Hg. To standardize BE for hemoglobin, the following formula was developed with improved in vivo accuracy, the standardized base excess (SBE):
SBE = 0.9287 X (HCO3 – 24.4 + 14.83 X [pH – 7.4])
Strong Ion approach to acid/base physiology
These classical descriptions of acid/base physiology often failed to account for acid/base findings in critically ill patients. An alkalosis was often noted in critically ill patients as their serum albumin level decreased, which could not be quantified by Henderson Hasselbalch or BE. Also, the “dilutional” acidosis frequently encountered after a large infusion of normal saline could not be explained by either of these 2 approaches to acid/base balance.
Both Henderson Hasselbalch and BE assume that the cations (Ca2+, Mg2+) and anions (Cl–, albumin, PO4–) in plasma remain unchanged in a patient with metabolic acidosis. Yet, in critically ill patients, these ions are known to be in dynamic flux. During the 1980s, Dr. Peter Stewart developed an acid/base theory (Strong Ion) using quantitative chemistry, which accounted for fluctuations of all the ions dissolved in plasma. Based on the requirements for electrical neutrality in any solution as any one of the concentrations of these ions changes, water must dissociate into H+ or OH– to balance the charge. The pH in this scheme is not a consequence of the ratio of acid to base in solution but determined by 3 independent variables:
Strong ion difference (SID) – Ions almost completely dissociated at physiologic pH.
SID = [Na+ + K+ + Ca2+ + Mg2+] – [Cl– + Lactate–]
(Ca2+ and Mg2+ are the concentrations of their ionized forms, Mg2+ X 0.7 = ionized Mg2+ concentration)
Total weak acid concentration (Atot) – Ions that can exist dissociated (A-) or associated (AH) at physiologic pH (buffers)
Atot = 0.325275 X [albumin] + 2 X [phosphate]
pCO 2 (mm Hg)
The Henderson Hasselbalch equation can be reformulated with variables from the Strong Ion Theory to give a more generalizeable solution to pH.
pH = pK1 ’ + log [SID] – Ka – [ATOT]/[Ka + 10–pH]
SPCO2
(K1’ is the equilibrium constant for the Henderson-Hasselbalch equation, Ka is the weak acid dissociation constant, and S is the solubility of CO2 in plasma.) See the image below.
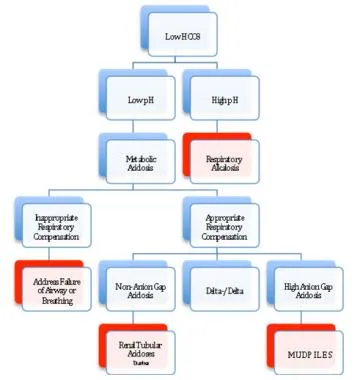
Once a metabolic acidosis is suspected by low bicarbonate concentration, an arterial blood gas analysis should be obtained. The low HCO3 level can be caused either by a primary metabolic acidosis or as the metabolic compensation for a respiratory alkalosis. The direction of the pH will separate metabolic acidosis (pH < 7.35) from a respiratory alkalosis (pH > 7.45).
The normal respiratory response (Kussmaul breathing) to a metabolic acidosis is a decrease in pCO2. This is given by the Winter’s equation:
PCO2 = 1.5 X (observed HCO3) + 8±2
(A quick rule of thumb: The PCO2 should approximate the last two digits of pH. For example, pH 7.25, PCO2 should be close to 25 mm Hg.)
Failure to have an appropriate respiratory response to metabolic acidosis represents a failure of airway and/or breathing, which must be addressed before any other workup commences.
Once an appropriate respiratory response for a metabolic acidosis has been established, the workup for the presence of unmeasured anions can progress by using the traditional anion gap, the delta-delta approach, or the strong ion gap. This allows the differential of metabolic acidosis to be narrowed and the appropriate therapy applied.
To differentiate between the causes of metabolic acidosis, one traditionally calculates the anion gap (AG), corresponding the presence of unmeasured anions.
AG = (Na+) – ([Cl–] + [HCO3–])
The anion gap allows for the differentiation of 2 groups of metabolic acidosis. Metabolic acidosis with a high AG is associated with the addition of endogenously or exogenously generated acids. Metabolic acidosis with a normal AG is associated with the loss of HCO3 from the kidney or GI tract, or the failure of the kidney to excrete H+.
The delta/delta concept allows for the partitioning of metabolic acidosis into an anion gap and a non-anion gap component, which can occur contemporaneously. The concept behind delta/delta is based on the assumption that for every increase in anion gap of 1 mmol/L above normal (12 mmol), serum HCO3– will drop by an equal amount.
Δ anion gap = Δ HCO3
If the delta HCO3– is greater than the delta anion gap, then a concomitant non-anion gap acidosis must exist along side the anion gap acidosis. One example would be a patient with a congenital renal tubular acidosis in diabetic ketoacidosis (DKA).
Stewart provides a replacement for the standard anion gap and delta/delta, which allows one to directly measure the amount of unmeasured anions in solution corrected for changes from normal of Ca2+, Mg2+, albumin, and phosphate. This is the Strong Ion Gap (SIG). All the strong ions are expressed in mEq/L, and only the ionized portions of Mg2+ and Ca2+ are considered (to convert total to ionized Mg2+, multiply by 0.7). Because of the complexity of the equation, several Internet resources are available to calculate the SIG. For example, The Stewart approach to acid-base is a good resource. The normal SIG is between 0 and 2. SIG has been shown to be better than blood lactate, pH, or injury severity scores in trauma patients and pediatric surgery patients as a predictor of mortality.
A report by Masevicius and Dubin stated, however, that the variables presumed to be independent in the Stewart approach are actually interdependent in various situations and that there is a lack of experimental evidence to show that water dissociates in response to SID changes. The report also contends that severe methodologic drawbacks exist in studies that have attempted to demonstrate that the Stewart approach is clinically superior to conventional methods for analyzing acid-base disorders, while the largest such study indicated that the Stewart approach can be used interchangeably with conventional techniques. Masevicius and Dubin concluded that the Stewart approach does not offer a significantly better method of understanding, diagnosing, and treating acid-base changes in critically ill individuals.

Prognosis
Because metabolic acidosis is a condition that occurs in response to a variety of disease states, the prognosis is directly related to the underlying etiology and the ability to treat or correct that particular disorder.
A study by Raikou indicated that in patients undergoing renal replacement therapy, an association exists between uncorrected severe metabolic acidosis (serum bicarbonate concentrations of below 20 mmol/L) and a 10-year risk for coronary heart disease of over 20%, as well as a high overall mortality rate.
A study by Park et al indicated that a high rate of metabolic acidosis occurs in kidney transplant recipients; a low serum total CO2 concentration (< 22 mmol/L) was found in about 30-70% of such patients with an estimated glomerular filtration rate of under 30 mL/min per 1.73 m2. The study also found evidence that metabolic acidosis may increase the likelihood of graft failure and mortality in kidney transplant recipients.
In a study of emergency department patients with acute kidney injury, Safari et al determined, through multivariate analysis, that metabolic acidosis is independently associated with mortality, along with sex, age over 60 years, blood urea nitrogen (BUN) concentration, hyperkalemia, cause of renal failure, and type of renal failure.
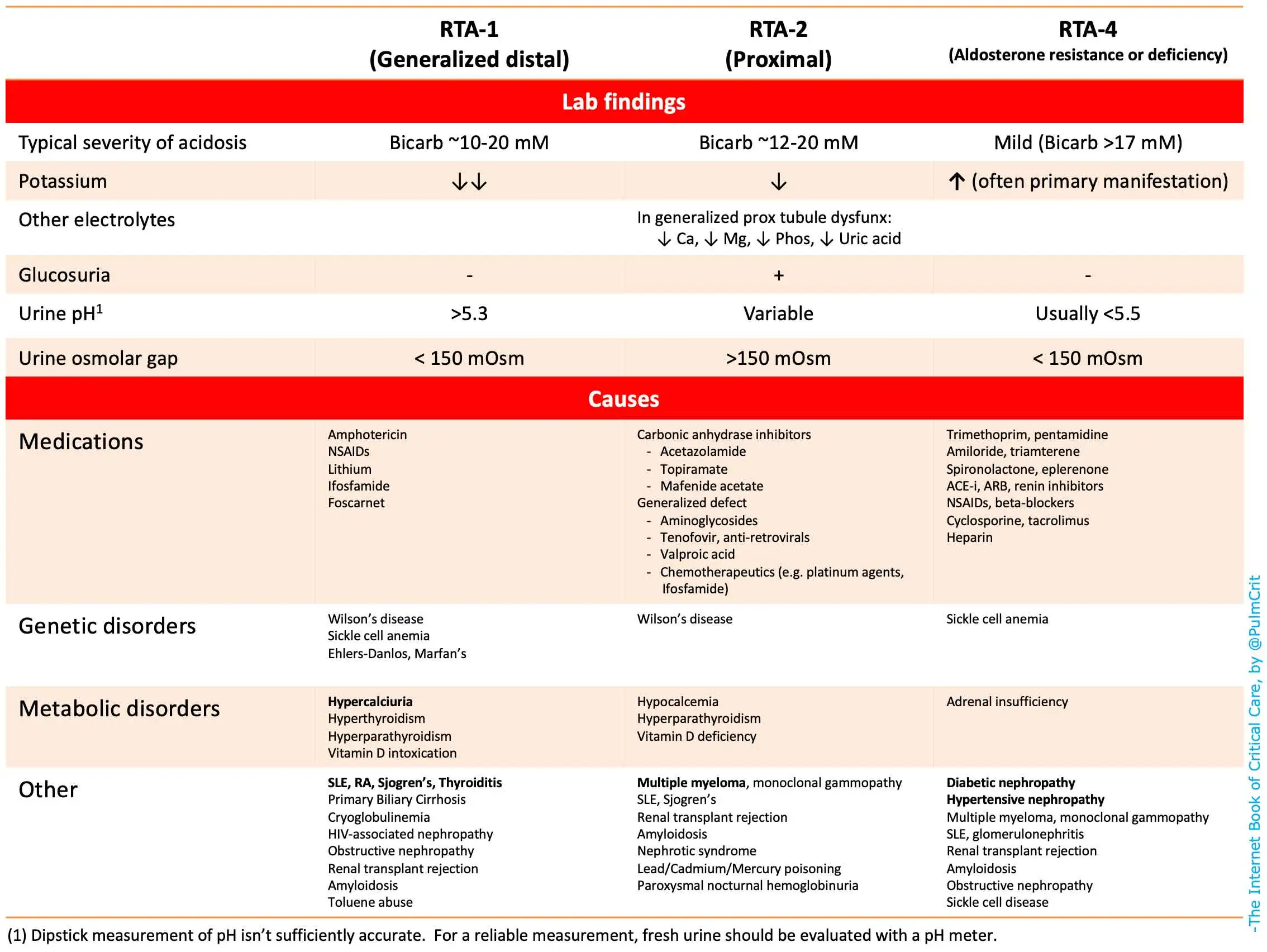

History
Metabolic acidosis can result in a variety of nonspecific changes in several organ systems, including, but not limited to, neurologic, cardiovascular, pulmonary, gastrointestinal, and musculoskeletal dysfunction. Symptoms are often a result of and specific to the underlying etiology of the metabolic acidosis.
Head, eyes, ears, nose, throat (HEENT) findings include the following:
Tinnitus, blurred vision, and vertigo can occur with salicylate poisoning.
Visual disturbances, dimming, photophobia, scotomata, and frank blindness can be seen in methanol intoxication.
Cardiovascular findings include the following:
Palpitations
Chest pain
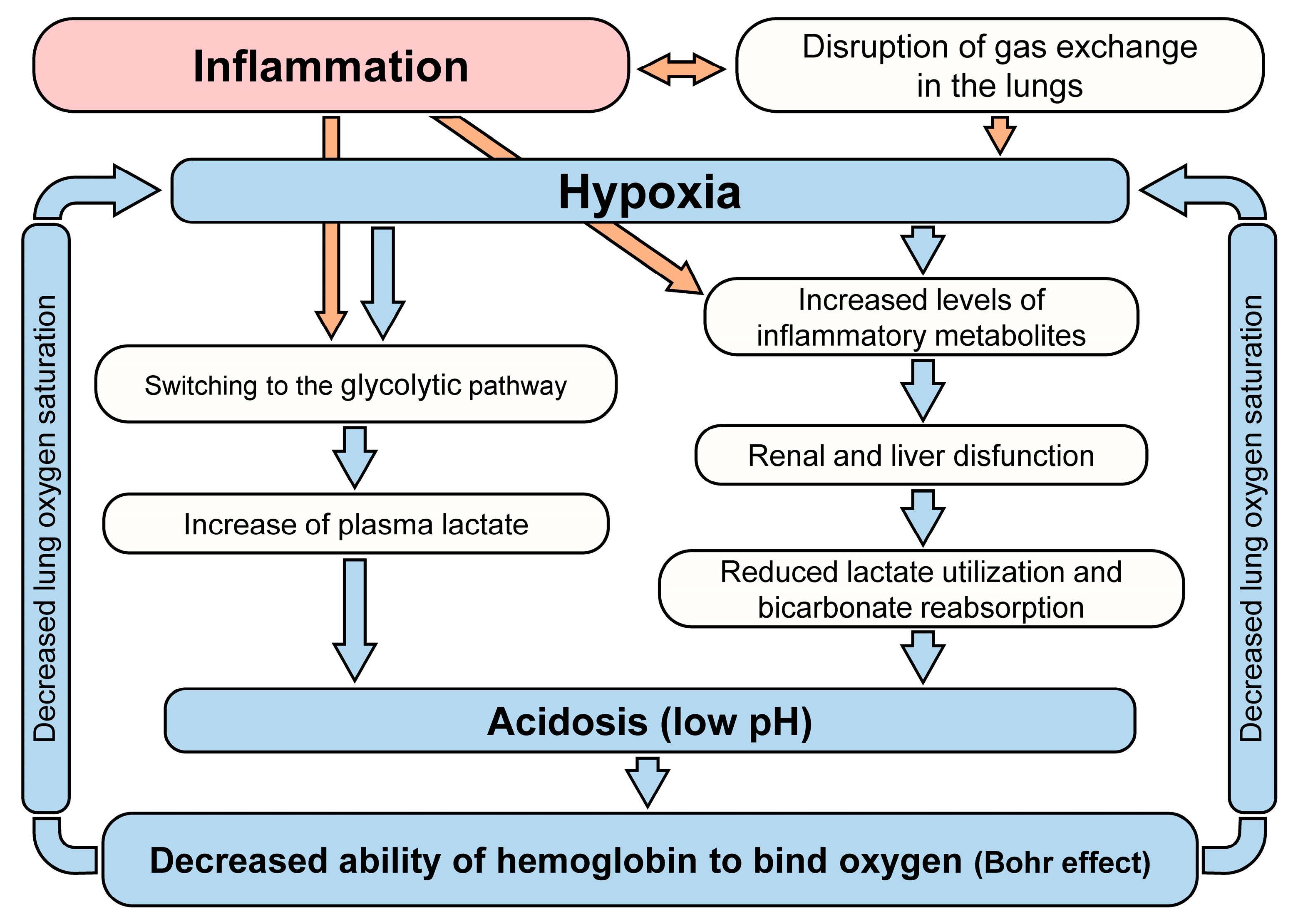
Neurologic findings include the following:
Headache
Visual changes
Mental confusion
Pulmonary findings include subjective dyspnea from the patient’s observation of hyperventilation.
GI findings include the following:
Nausea and vomiting
Abdominal pain
Diarrhea
Polyphagia
Musculoskeletal findings include the following:
Generalized muscle weakness
Bone pain
Physical
Neurologic
Cranial nerve palsies may occur with ethylene glycol intoxication.
Retinal edema may be seen in methanol ingestions.
Lethargy, stupor, and coma may occur in severe metabolic acidosis, particularly when it is associated with a toxic ingestion.
Cardiovascular
Severe acidemia (ie, pH < 7.10) can predispose a patient to potentially fatal ventricular arrhythmias, and it can reduce cardiac contractility and the inotropic response to catecholamines, resulting in hypotension and congestive heart failure.
Pulmonary
Patients with acute metabolic acidosis demonstrate tachypnea and hyperpnea as prominent physical signs.
Kussmaul respiration, an extremely intense respiratory effort, may be present.
Hyperventilation, in the absence of obvious lung disease, should alert the clinician to the possibility of an underlying metabolic acidosis.
Musculoskeletal
Chronic metabolic acidosis (eg, uremia, renal tubular acidosis [RTA]) is associated with substantial bone disease from bone buffering of calcium carbonate.
Causes
Causes of inability to excrete the dietary H+ load are as follows:
Renal failure – Diminished NH4+ production
Hypoaldosteronism – Type 4 RTA
Diminished H+ secretion – Type 1 (distal) RTA
Causes of increased H+ load include the following:
Lactic acidosis – Numerous causes, including circulatory failure, drugs and toxins, and hereditary causes
Ketoacidosis – Diabetes, alcoholism, and starvation
Ingestions – Salicylates, methanol, ethylene glycol, isoniazid, iron, paraldehyde, sulfur, toluene, ammonium chloride, phenformin/metformin, and hyperalimentation fluids
GI HCO3– loss is caused by the following:
Diarrhea
Pancreatic, biliary, or intestinal fistulas
Ureterosigmoidostomy
Cholestyramine
Renal HCO3– loss may be caused by type 2 (proximal) RTA.
Differential Diagnoses
Acute Kidney Injury (Renal Failure) in Emergency Medicine
Dialysis Complications of Chronic Renal Failure
Laboratory Studies
Arterial blood gas analysis
A low HCO3 level found on an automated sequential multiple analyzer (SMA) (eg, serum chemistries) is often the first clue to the presence of a metabolic acidosis; however, it cannot be the only consideration in the diagnosis of metabolic acidosis. A low HCO3 level can be caused by metabolic acidosis, a metabolic compensation of a respiratory alkalosis, or a laboratory error.
The HCO3 level that is calculated by the arterial blood gas (ABG) machine, which uses the Henderson-Hasselbalch equation, represents a more accurate measure of the plasma HCO3 level than the SMA measurement. It is suggested that the HCO3level that is determined from the ABG be used in the anion gap calculation instead of the HCO3 level found using the SMA.
Measurement of pH and PCO2 by ABG in a patient with a low HCO3 level makes it possible to differentiate a metabolic compensation of a respiratory alkalosis from a primary metabolic acidosis. Measurement of PCO2 also makes it possible to judge the appropriateness of respiratory compensation of a metabolic acidosis, and to detect respiratory acidosis, which is signified by an elevated PCO2 level.
Oxygenation does not affect the acid-base status of a patient and generally should not be part of the discussion unless severe hypoxia is leading to ischemia. In that case, measurement of PO2 can identify severe hypoxia as a precipitant of lactic acidosis.
ABGs also measure base excess/base deficit (BE/BD), which is the best indicator of the degree of acidosis/alkalosis. BE/BD is measured by gauging the amount of acid or base that is required to titrate the patient’s blood sample to a pH of 7.40, given a PCO2 level of 40 mm Hg at 37 degrees Celsius. BE/BD is a more accurate reflection of the body’s state, and it is recommended over calculations using the HCO3 level.
Serum chemistry
Sodium, potassium, chloride, and bicarbonate levels are used in the calculation of serum anion gap (SIG). Phosphate and magnesium levels, as well as serum albumin levels, are used to calculate the SIG.
Hyperkalemia often complicates metabolic acidosis. It commonly is seen with inorganic acidosis (ie, non-AG). Diabetic ketoacidosis (DKA) often presents with hyperkalemia that does not parallel the acidosis; in this case, hyperkalemia results from insulin deficiency and the effects of hyperosmolality. Lactic acidosis and other forms of organic acidosis generally do not present with a significant potassium shift.
Glucose level is commonly elevated in DKA, and it may be low, normal, or mildly elevated in alcoholic ketoacidosis.
The BUN and creatinine levels are elevated in uremic acidosis.
CBC count
An elevation of the WBC count is a nonspecific finding, but it should prompt consideration of septicemia, which causes lactic acidosis.
Severe anemia with compromised O2 delivery may cause lactic acidosis.
Urinalysis
A urine pH is normally acidic at < 5.0. In acidemia, the urine normally becomes more acidic. If the urine pH is above 5.5 in the face of acidemia, this finding is consistent with a type I RTA. Alkaline urine is typical in salicylate poisoning.
Ethylene glycol toxicity may present with calcium oxalate crystals, which appear needle shaped, in the urine.
Imaging Studies
If iron ingestion is suspected, perform imaging studies on the abdominal area, including the kidneys, ureters, and bladder.
Other Tests
Anion gap (AG)
Calculation of the AG is often helpful in the differential diagnosis of metabolic acidosis. [6] The AG is equal to the difference between the plasma concentrations of the measured plasma cation (ie, Na+) and the measured anions (ie, chloride [Cl–], HCO3–). It exists because standard electrolyte panels do not measure all the anions present in the serum.
AG calculation = (Na+) – ([Cl–] + [HCO3–])
A normal AG is traditionally listed as 8-16 mEq/L, with an average value of 12. This value may vary, depending on the instrumentation used to measure electrolyte levels, and recent data suggest a normal range of 5-11 mEq/L. Some authors add K+to measured cations; then, the traditional normal range is 12-20 mEq/L. The anion gap allows for the differentiation of 2 groups of metabolic acidosis. Metabolic acidosis with a high AG is associated with the addition of endogenously or exogenously generated acids. Metabolic acidosis with a normal AG is associated with the loss of HCO3 or the failure to excrete H+ from the body.
High AG warrants the following:
Lactic acidosis – Lactate, D-lactate
Ketoacidosis – Beta-hydroxybutyrate, acetoacetate
Renal failure – Sulfate, phosphate, urate, and hippurate
Ingestions – Salicylate, methanol or formaldehyde (formate), ethylene glycol (glycolate, oxalate), paraldehyde (organic anions), sulfur (SO4–), phenformin/metformin
Pyroglutamic acidemia (5-oxoprolinemia)
Massive rhabdomyolysis (release of H+ and organic anions from damaged muscle)
Several mnemonics are used to prompt recall of the differential diagnosis of high anion gap acidosis. Two, neither of which is completely comprehensive, are as follows:
MUDPILES: M-methanol; U-uremia; D-DKA, AKA; P-paraldehyde, phenformin; I-iron, isoniazid; L-lactic (ie, CO, cyanide); E-ethylene glycol; S-salicylates
DR. MAPLES: D-DKA; R-renal; M-methanol; A-alcoholic ketoacidosis; P-paraldehyde, phenformin; L-lactic (ie, CO, HCN); E-ethylene glycol; S-salicylates
Normal AG (ie, hyperchloremic acidosis) indicates the following:
GI loss of HCO3–, diarrhea
Pancreatic fistula
Renal HCO3– loss – Type 2 (proximal) RTA
Renal dysfunction
Some cases of renal failure
Hypoaldosteronism (ie, type 4 RTA)
Hyperventilation
Ingestions – Ammonium chloride, acetazolamide, hyperalimentation fluids, some cases of ketoacidosis, particularly during treatment with fluid and insulin
The AG can rise because of increases in unmeasured anions or decreases in unmeasured cations (eg, hypokalemia, hypocalcemia, hypomagnesemia). AG can also increase, secondary to an increase in albumin or an increase in negative charges on albumin, which is caused by alkalosis.
AG can be decreased by an increase in unmeasured cations (eg, hyperkalemia, hypercalcemia, hypermagnesemia, lithium intoxication, high immunoglobulin G [IgG] levels), or by a decrease in unmeasured anions (eg, hypoalbuminemia).
Finally, laboratory errors can also affect the AG. Hyperproteinemia, hyperlipidemia, and hyperglycemia resulting in underestimation of serum sodium level can falsely depress AG. In addition, bromide intoxication can be mistaken for Cl–, which can result in an inappropriate depression of the AG.
The osmolal gap is the measured plasma osmolality minus calculated osmolality. The serum osmolality is composed of all osmotically active substances including ionic and nonionic substances such as serum ions, glucose, and BUN. Other substances such as alcohols, excess serum lipids and proteins, and delivered substances such as mannitol all contribute to the serum osmolality. The calculated osmolality is 2 X plasma [Na+] + [glucose]/18 + BUN/2.8.
Normal osmolal gap is 10-15.
Metabolic acidosis with elevated osmolal gap indicates methanol and ethylene glycol ingestions.
Ketone level
Elevations of ketones indicate diabetic, alcoholic, and starvation ketoacidosis.
The nitroprusside test is used to detect the presence of ketoacids in the blood and the urine. This test only measures acetoacetate and acetone; therefore, it may underestimate the degree of ketonemia and ketonuria because it will not detect the presence of beta-hydroxybutyrate (BOH). This limitation of the test can be especially problematic in patients with ketoacidosis who cannot convert BOH to acetoacetate because of severe shock or liver failure.
An assay for BOH is unavailable in some hospitals. An indirect method to circumvent this problem is to add a few drops of hydrogen peroxide to a urine specimen. This enzymatically will convert BOH into acetoacetate, which will be detected by the nitroprusside test.
Serum lactate level
For a complete discussion of the differentials of lactic acidosis, refer to Lactic Acidosis.
The normal plasma lactate concentration is 0.5-1.5 mEq/L.
Lactic acidosis is considered present if the plasma lactate level exceeds 4-5 mEq/L in an acidemic patient.
Salicylate levels
Therapeutic salicylate levels range up to 20-35 mg/dL.
Plasma levels exceeding 40-50 mg/dL are in the toxic range.
Plasma levels provide some information as to the severity of intoxication: 40-60 mg/dL is considered mild; 60-100 mg/dL is moderate; and greater than 100 mg/dL is considered severe.
Iron levels
Iron toxicity is associated with lactic acidosis.
Iron levels greater than 300 mg/dL are considered toxic.
Electrocardiography
An ECG may be used to detect abnormalities that result from the effects of electrolyte imbalances (eg, hyperkalemia).
Emergency Department Care
The initial therapeutic goal for patients with severe acidemia is to raise the systemic pH above 7.1-7.2, a level at which dysrhythmias become less likely and cardiac contractility and responsiveness to catecholamines will be restored.
Metabolic acidosis can be reversed by treating the underlying condition or by replacing the bicarbonate. The decision to give bicarbonate should be based upon the pathophysiology of the specific acidosis, the clinical state of the patient, and the degree of acidosis.
Treating the underlying conditions in high AG states usually is sufficient to reverse the acidosis. Treatment with bicarbonate is unnecessary, except in extreme cases of acidosis when the pH is less than 7.1-7.2. For all cases of diabetic ketoacidosis (DKA), the role of bicarbonate is controversial, regardless of the pH or bicarbonate level.
In hyperchloremic acidosis, the central problem is with the reabsorption or regeneration of bicarbonate. In these conditions, therapy with bicarbonate makes physiologic sense and is prudent in patients with severe acidosis.
Caution with bicarbonate therapy is indicated because of its potential complications, including the following:
Volume overload
Hypokalemia
CNS acidosis
Hypercapnia
Tissue hypoxia via leftward shift of hemoglobin-oxygen dissociation curve
Alkali stimulation of organic acidosis (lactate)
Overshoot alkalosis
A British study, by Misra et al, indicated that emergency hospital admissions for DKA increased during the coronavirus disease 2019 (COVID-19) pandemic. The investigators looked at the periods from March 1 to June 30, 2020 (first wave of the pandemic); July 1 to Oct 31, 2020 (post–first wave period); and Nov 1, 2020, to Feb 28, 2021 (second wave of the pandemic), comparing them to equivalent periods between March, 2017, and February, 2020. They found that emergency admissions for DKA rose 6%, 6%, and 7%, respectively. However, the changes in emergency DKA admissions varied according to the type of diabetes considered. During the first wave, for example, such admissions in persons with preexisting type 1 diabetes actually fell by 19%, while in those with preexisting type 2 diabetes and patients with newly diagnosed diabetes, the admissions increased by 41% and 57%, respectively.
Consultations
Metabolic acidosis secondary to ingestions (eg, salicylate, methanol, ethylene glycol) often requires dialysis therapy, and a nephrologist should be consulted early in the case management. Toxicologic consultation should also be considered in such cases. Dialysis is the preferred treatment for patients with significant metabolic acidosis in the setting of renal failure.
A retrospective, cross-sectional study by Nogi et al indicated that intermittent hemodialysis can effectively lead to hemodynamic and respiratory stabilization in patients with septic shock complicated by metabolic acidosis. Following the procedure, mean arterial pressure in the study’s patients was found to have increased, minute ventilatory volume to have decreased, norepinephrine requirement to have decreased, bicarbonate level to have increased, and pH to have increased.
Medication Summary
Many drugs may be used in the management of a patient with metabolic acidosis. They range from antibiotics for septic shock to toxin antidotes. These agents are discussed in detail under the specific articles for the disease. Bicarbonate is an agent that is considered across the numerous differentials of metabolic acidosis. Its use generally is limited to severe cases of acidosis (pH < 7.1-7.2).
Alkalinizing agent
Class Summary
This agent is used in the treatment of metabolic acidosis.
Sodium bicarbonate (Neut)
Bicarbonate ion is produced when it dissociates and neutralizes the hydrogen ions and raises urinary and blood pH.
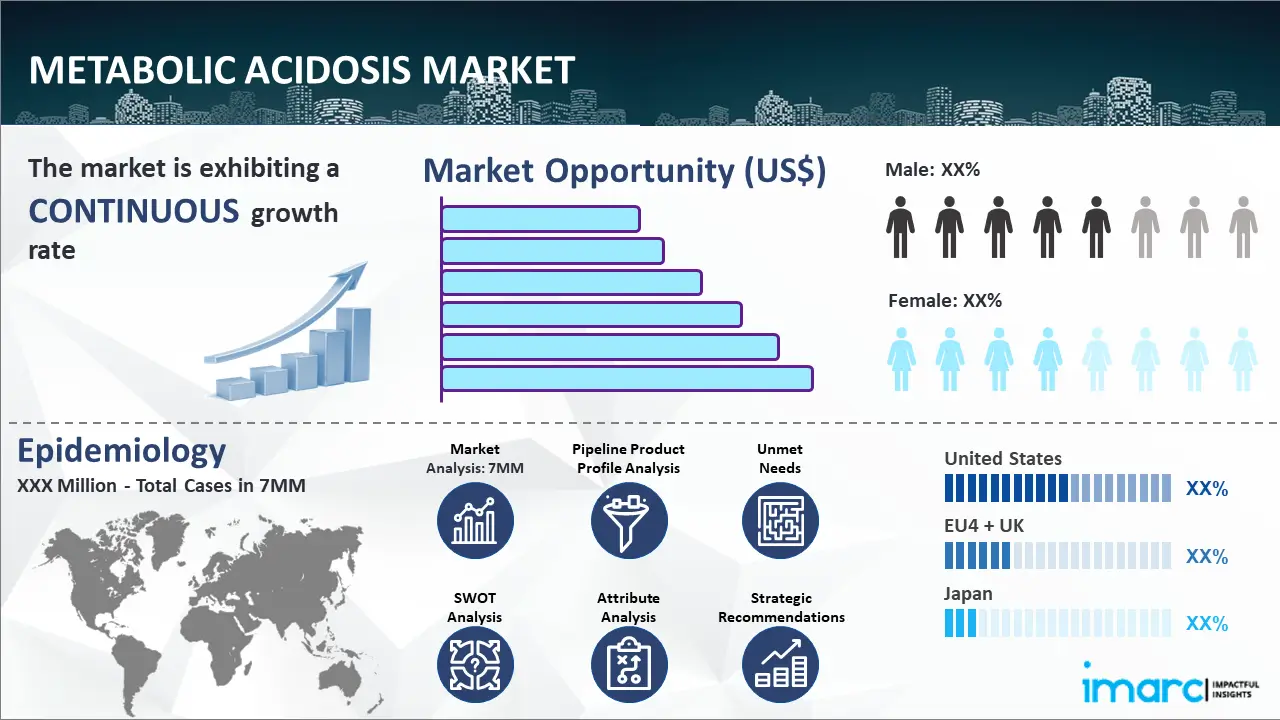
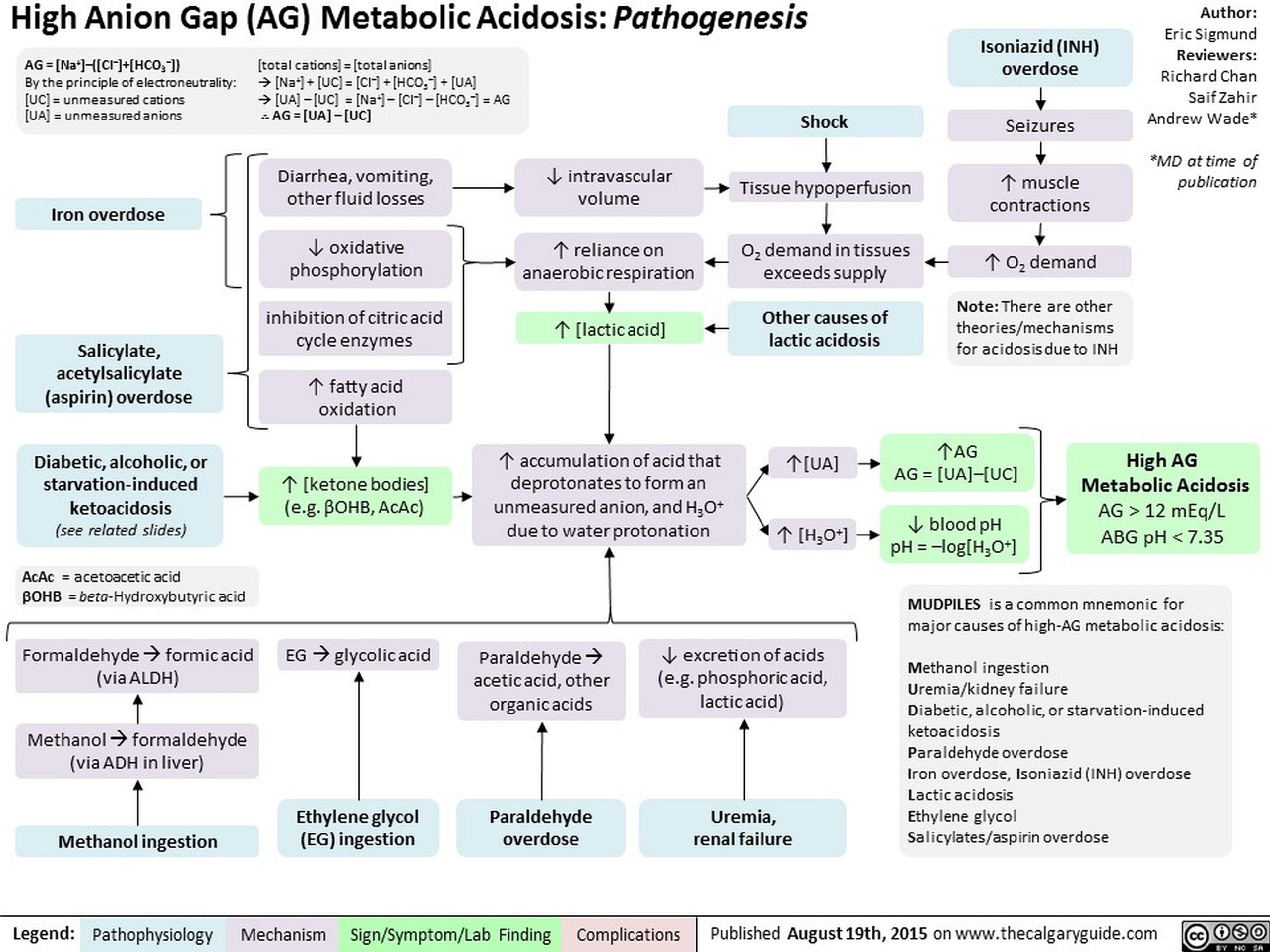