Overview
This is a four-part series covering gastrointestinal physiology.
- Part I – Overview of the gastrointestinal system & the oral cavity.
- Part II – Digestion: stomach & control of digestion.
- Part III – Secretion: pancreas & hepatobiliary system.
- Part IV – Absorption: small intestine.
The small intestine is the main site of absorption within the gastrointestinal tract. It is approximately 3-6m in length, extending from the pylorus of the stomach to the ileocaecal valve, and is divided into the:
- Duodenum: runs from the pylorus of the stomach to duodenojejunal junction in a C-shape. It is the shortest part of the small intestine, typically 25cm in length.
- Jejunum: runs from the duodenojejunal junction to the ileum, variable in length but typically composes the proximal two-fifths of the small intestine beyond the duodenum.
- Ileum: runs from the ileum to the ileocaecal valve, variable in length but typically composes the distal three-fifths of the small intestine beyond the duodenum. The most distal segment is often referred to as the terminal ileum.
Digestive contents are propelled towards the large intestines by peristalsis. The small intestine is well adapted for absorption due to the large surface area and specialised enterocytes.
Surface area
The small intestines are highly folded increasing the surface area available for absorption.
Three special modifications greatly increase the surface area of the small intestines:
- Valvulae conniventes
- Villi
- Microvilli
The total surface area of the mucosa of the small intestines is disputed. It has often been quoted as 300m2 or the size of a tennis lawn. However, more recent reviews have revised this estimate to closer to 30m2.
Valvulae conniventes
The inner surface of the intestines contains numerous circular folds visible at endoscopy, which are the valvulae conniventes. These folds increase the surface area by around 1.6 times. They can be seen as a line running across the lumen on an abdominal X-ray. This is one of the distinguishing features between small and large bowel (large bowel have haustra, seen as incomplete lines from the edges of the large bowel wall on X-ray).
Villi
Lining the intestinal mucosa are microscopic finger-like projections called villi. Villi have a lining of enterocytes joined laterally by tight junctions. The luminal border of these enterocytes has numerous transport mechanisms and digestive enzymes to aid in the absorption process.
Each villus is formed by a core of connective tissue from the lamina propria and further supplied by vascular and lymphatic tissue. Each villus contains a vascular network including an arteriole, capillary bed and venule. Together these help in the absorption of nutrients, which can be passed towards the liver via the portal system.
A central lymphatic duct can also be found within each villus. This terminal duct, called a lacteal, is important in the absorption and distribution of fats.
Microvilli
Microvilli are minute hair-like structures that are found on the surface of enterocytes lining the villi, a microscopic extension of the plasma membrane. Each epithelial cell may contain up to 3000-6000 microvilli, which helps form the intestinal brush-border.
Together villi and microvilli increase the mucosal surface of the small intestines by 60-120 times.
Crypts of Lieberkühn
The crypts of Lieberkühn are tubular pits that open onto the epithelium of the small intestines.
The crypts of Lieberkühn (also termed intestinal glands) are situated between the villi within the intestinal mucosa. The crypts contain numerous cells lines:
- Stem cells
- Enterocytes
- Paneth cells
- Endocrine cells
- Goblet cells
Stem cells are important in the regeneration of the epithelial lining of the intestines. They differentiate into enterocytes and goblet cells as they migrate towards the top of the villus over 3-6 days before undergoing apoptosis.
Paneth cells have important functions in host defence and the secretion of antimicrobial peptides. Endocrine cells are critical for neurohormonal control of the digestive tract and goblet cells secrete mucus.
Enterocytes are predominantly responsible for the production of intestinal fluids in the small intestine. Approximately 1-2 litres of intestinal fluid is secreted each day.
Functions
The four main functions of the small intestines are secretion, digestion, absorption and motility.
Secretion
The small intestines secrete up to 1-2 litres of digestive juices per day. These digestive juices are called the succus entericus, and are produced by enterocytes.
Digestion
Digestion refers to the large, complex food molecules into smaller, typically water-soluble, components that can be absorbed. It may be mechanical (e.g chewing, peristalsis) or chemical (mediated by enzymes)
The small intestines are important for the digestion of lipids, carbohydrates and proteins. Enterocytes contain a number of key enzymes on their luminal surface, which helps break large molecules into smaller fragments or single units that can be absorbed.
- Carbohydrates: are broken down by disaccharidases such as maltase, sucrase and lactase. These enzymes break disaccharides into single monosaccharide units. Sucrase converts sucrose into glucose and fructose, maltase converts maltose into two glucose molecules and lactase converts lactose into galactose and glucose.
- Proteins: are acted upon by pancreatic proteases. Enterokinase converts trypsinogen into the active enzyme trypsin. Trypsin is then able to activate further trypsinogen and chymotrypsinogen (to chymotrypsin). Trypsin, chymotrypsin along with elastase convert proteins to polypeptides. Carboxypeptidases (also released by the pancreas) can cleave individual amino acids from small polypeptides. Digestion also occurs on the luminal surface by peptidases on the luminal membrane of enterocytes. They produce small peptides (e.g. di-, tri-peptides) and single amino acids.
- Lipids: the small intestines is the major site of fat digestion. Bile, released by the hepatobiliary system, emulsifies fa. Pancreatic lipase breaks down triglycerides to free fatty acids and monoglycerides. Micelles are formed with an outer lining of bile salts and products of fat digestion internally, that can be absorbed by enterocytes.
Absorption
Absorption is the primary function of the small intestines. It describes the transfer of nutrients from our diet into the circulation. We have specialised mechanisms for the absorption of many substances including carbohydrates, proteins, lipids, iron and calcium. These processes are all described in further detail below.
Motility
The motility of the small intestines is divided into two important mechanisms:
- Segmentation: is the process of ring-like contractions of the small intestines that help to mix and propel intestinal content. These contractions occur due to oscillating circular muscle contraction. They are controlled by the basic electrical rhythm (BER). The BER refers to oscillations in membrane potential that bring the muscle closer and further from threshold potential.
- Migrating motility complex (MMC): during times of fasting, segmentation stops and the MMC takes over, which is often described as the ‘intestinal housekeeper’. Peristaltic waves are generated, initially in the stomach, and cause a wave of depolarisation throughout the digestive tract, which moves intestinal content forward. Over 100-150 minutes the remaining intestinal content is swept towards the colon. The cycle can then be repeated once finished. The MMC is regulated by the hormone motilin.
Absorption principles
Most nutrients consumed within the diet are absorbed unopposed by the intestines.
Most absorption takes place within the duodenum and jejunum. Up to 50% of the small intestines can be removed with little effect on overall absorption. Only calcium and iron absorption is regulated depending on our bodies’ requirements.
The process of absorption of dietary molecules occurs via transepithelial transport, which is the movement of molecules across the entire enterocyte. Molecules may either move passively down their electrochemical gradient, or the electrochemical gradient must be established using energy in the form of ATP (active transport).
Active transport requires the generation of an electrochemical gradient. Typically, a sodium/potassium pump on the basolateral membrane moves sodium out of the cell and potassium into the cell with the utilisation of ATP. This establishes an electrochemical gradient for the movement of sodium into the cell across the luminal membrane. Sodium then co-transports molecules (e.g. glucose/amino acids) across the luminal membrane.
Once inside the cells, sodium is actively pumped out by the basolateral sodium/potassium pump, and products of digestion can be absorbed via facilitated diffusion.
Protein absorption
Proteins can be absorbed from the diet as amino acids or small peptide fragments.
Amino acids
Sodium/potassium ATPase pumps on the basolateral membrane generate an electrochemical gradient. This allows the movement of sodium down its concentration gradient via a symporter on the luminal membrane.
The symporter is coupled with the movement of amino acid molecules thereby facilitating absorption. This process is secondary active transport. Once inside the cell, amino acids can be absorbed into the portal circulation by a variety of different passive carriers on the basolateral membrane.
Peptide fragments
Sodium/potassium ATPase pumps on the basolateral membrane generate an electrochemical gradient. Sodium then moves into the enterocyte by an antiporter, which shuttles hydrogen ions out of the cell on the luminal membrane.
The movement of hydrogen ions out of the cell generates a second electrochemical gradient. Hydrogen then moves into the cell via a symporter on the luminal membrane. This symporter is coupled with the movement of peptide fragments. This process is tertiary active transport.
Once inside the enterocyte, these peptide fragments are broken into amino acids by intracellular peptidases before absorption into the portal circulation.
Carbohydrate absorption
The absorption of carbohydrates is dependent on the type of monosaccharide.
Glucose/galactose
Sodium/potassium ATPases on the basolateral membrane generate an electrochemical gradient. This allows sodium to moves down its electrochemical gradient into the enterocyte through the sodium-glucose transporter. The movement of sodium into the cell is coupled with the movement of glucose/galactose. This process is secondary active transport.
Glucose/galactose are then passively absorbed by facilitated diffusion across the GLUT-2 transporter on the basolateral membrane.
Fructose
Fructose is transported into the enterocyte by facilitated diffusion through the GLUT-5 transporter on the luminal membrane. Once inside the cell, fructose moves into the portal circulation via the GLUT-2 transporter on the basolateral membrane. This process is passive diffusion.
Lipid absorption
The absorption of lipids is dependent on the emulsification of fats by bile salts.
Pancreatic lipase breaks triglycerides into free fatty acids and monoglycerides. These structures are then encased by bile salts and lecithin to form small molecules called micelles. Micelles carry products of fat digestion and lipid-soluble components (e.g. cholesterol, fat-soluble vitamins) to the surface of enterocytes.
Free fatty acids and monoglycerides at the centre of the micelle freely diffuse across the luminal membrane into enterocytes. Inside the enterocyte, fatty acids and monoglycerides reform to make triglycerides. Triglycerides are then coated with a layer of lipoprotein created by the endoplasmic reticulum. This forms the smallest lipoprotein; a chylomicron.
Chylomicrons are then excreted into the central lacteal of the villi and transported through the lymphatic system. At the junction of the lymphatic duct with the subclavian vein, chylomicrons join the systemic circulation and are taken to muscle and adipose tissue where free fatty acids can be liberated. The remnant chylomicron is then taken to the liver and broken down. This transport of lipids from the intestines to the liver is called the exogenous pathway.
Iron absorption
Iron absorption is critical for the formation of haemoglobin.
Iron ingested within the diet may be in the form of ferrous (Fe2+), ferric (Fe3+) or haem. Only ferrous iron and haem can be absorbed by the intestines. There are mechanisms at the luminal surface, which help to reduce ferric iron into ferrous to enable absorption.
Ferrous iron and haem are absorbed across the luminal membrane in different transporters:
- Ferrous iron: transported by divalent metal transporter 1 (DMT).
- Haem: transported by haem carrier protein 1.
Once inside the cell, iron can be converted into a cytosolic store of ferritin. Iron that is not required by the body remains in enterocytes as ferritin. From here it is either utilised or lost in the faeces during epithelial regeneration. Iron that is needed is absorbed across a basolateral transporter called ferroportin. Iron absorption across ferroportin can be regulated by the molecule hepcidin. This molecule is secreted by the liver when iron levels are high.
Binding of hepcidin to ferroportin leads to the lysosomal destruction of the receptor preventing iron absorption. Ferroportin can also be found in the reticuloendothelial system on the plasma membrane of macrophages. Once iron exits enterocytes it is transported within the blood by the molecule transferrin. Transferrin is produced by the liver.
Calcium absorption
Calcium is an essential vitamin for bones and cellular processes.
Calcium is initially absorbed down its concentration gradient across the TRPV6 receptor on the luminal surface of enterocytes. It is then transported across the cell to the basolateral membrane by the molecule calbindin.
Calcium can then be absorbed into the bloodstream at the basolateral membrane by two different mechanisms:
- Calcium ATPase pump: primary active transport.
- Sodium/calcium antiporter: secondary active transport.
Vitamin D ( a fat-soluble vitamin), once activated, enhances every stage of this process to aid with calcium absorption from the small intestines. It binds to nuclear vitamin D receptors that induce gene transcription. The gene transcription products are molecules important in calcium absorption (e.g. calbindin, TRPV6).
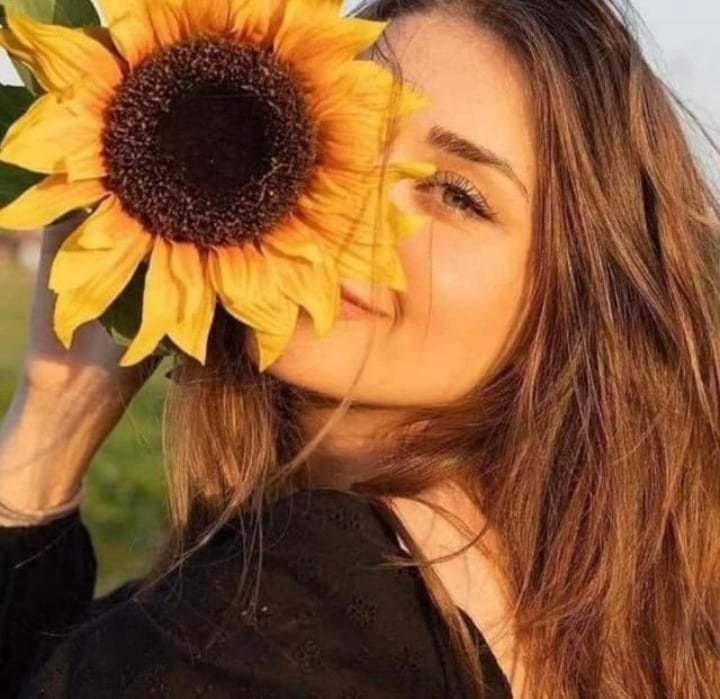